Scientist find a loophole in Heisenberg's uncertainty principle
Weird quantum behavior can exist at large scales too
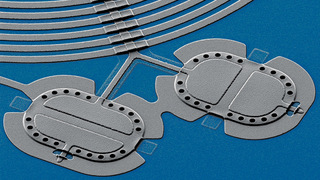
Quantum mechanics has brought its fair share of disquieting revelations, from the idea that objective reality is an illusion to the realization that objects can be in two states at once (both dead and alive, for instance). Such freaky quantum behavior doesn’t end when small objects become big — it’s just that our senses and our instruments aren’t able to detect it. Now, by banging on two sets of tiny drums, two teams of physicists have brought the scale at which we can observe quantum effects into the macroscopic realm.
The findings demonstrate a bizarre quantum effect called "entanglement" on a much larger scale than previously seen, as well as describing a way to use this effect — when particles remain connected to one another even if separated by great distances — to evade pesky quantum uncertainty. This knowledge could be used to probe quantum gravity and design quantum computers with calculative powers far beyond classical devices, according to researchers.
Physicists have long wondered at what scale weird quantum phenomena give way to our more familiar and predictable macroscopic world, mostly because there’s no hard and fast rule that says such phenomena ever should — they just become increasingly less observable as things scale up.
Related: 12 stunning quantum physics experiments
Or at least they used to. New experiments from two separate teams of researchers have made the leap from observing quantum entanglement between individual atoms to observing it between micron-sized aluminum membranes — or “drums” — made of roughly 1 trillion atoms each.
At its simplest, entanglement describes the idea that two particles can have an intrinsic connection that persists no matter how far apart they are. The particles are ethereally coupled: measure something about one particle, such as its position, and you’ll also glean information about the position of its entangled partner; make a change to one particle and your actions will teleport a corresponding change to the other, all at speeds faster than the speed of light.
The scientists in the first experiment, which took place at the U.S. National Institute of Standards and Technology (NIST) in Boulder, Colorado, placed tiny drums, each around 10 micrometers long, on a crystal chip, before supercooling them to near absolute zero. With the drums chilled, the chances of them interacting with something outside of the system was dramatically reduced, enabling the scientists to coax the drums into an entangled state, vibrating in sync as they were hit with regular pulses of microwaves.
"If you analyze the position and momentum data for the two drums independently, they each simply look hot," co-author John Teufel, a physicist at NIST, said in a statement, referring to the fact that particles vibrate more the hotter they get. "But looking at them together, we can see that what looks like random motion of one drum is highly correlated with the other, in a way that is only possible through quantum entanglement."
The researchers measured the extent of the drums’ entanglement by looking at how matched up their amplitudes — their maximum distances from their resting positions — were as they wobbled up and down by roughly the height of a single proton. The researchers saw that the drums vibrated in a highly synchronized way — when one drum had a high amplitude, the other had a low amplitude, and their velocities were exactly opposite values.
"If they have no correlations and they are both perfectly cold, you could only guess the average position of the other drum within an uncertainty of half a quantum of motion," said Teufal, referring to the discrete chunks or “quanta” that quantum objects like the drum will vibrate in. "When they are entangled, we can do better, with less uncertainty. Entanglement is the only way this is possible." The two large-scale vibrating drums appear to be two individual objects, but they are connected by spooky quantum entanglement.
The NIST researchers want to use their drum system to build nodes, or network end-points, in quantum networks, alongside adapting them for problems that need unprecedented levels of precision, like the detection of gravity when it acts on the smallest scale.
A second team of researchers, led by Mika Sillanpää at Aalto University in Finland set out to use their own quantum drum system to sidestep one of quantum physics’ strictest rules — the Heisenberg uncertainty principle.
First introduced by the German physicist Werner Heisenberg in 1927, the principle sets a hard limit to the absolute accuracy we can obtain when measuring some of the physical properties of a particle. It enshrines the idea that at its smallest, most fundamental level the universe is a fuzzy and unpredictable beast, never permitting complete information about it to be known.
You cannot know both a particle’s position and its momentum with absolute accuracy, for instance. Want to know exactly where an electron is located? You can measure it repeatedly to build up some certainty. But the more you do that, the more you interact with it, changing its momentum. The same thing happens the other way around. Certainty in the quantum world is a tradeoff — in a realm where objects exist more as clouds of probabilities, becoming more sure about one of their properties means becoming less sure about another.
But the second team of researchers found a way around this. By hitting their quantum drum continuously with photons, or light particles, like they would a snare drum, the researchers were able to tune their drums into an entangled state. Then, instead of measuring each individual drum’s position and momentum, the researchers treated the entangled drums as if they were a single, combined drum, and measured the imaginary drum’s position without affecting its velocity.
"The quantum uncertainty of the drums' motion is canceled if the two drums are treated as one quantum-mechanical entity," lead author Laure Mercier de Lepinay, a postdoctoral researcher at Aalto University in Finland, said in a statement.
This opens up a whole new range of possibilities for measurements to be made at the tiniest of scales without any information being lost, and, given the continuous way the measurement is taken, for their new quantum sensors to monitor constantly evolving, miniscule systems. The researchers hope that their entangled drums will be sensitive enough to measure the tiny distortions in space created by gravitational waves and dark matter, as well as being used to link together quantum networks, which use entangled objects much like their drums as relays.
Both experiments also confront us with the reality of our closeness to the quantum world, which — despite ostensibly farfetched thought experiments that summon up half-dead, half-alive cats — bleeds into our own in far subtler ways than we might think.
The first and the second teams both published their findings May 7 in the journal Science.
Originally published on Live Science.
Live Science newsletter
Stay up to date on the latest science news by signing up for our Essentials newsletter.
Ben Turner is a U.K. based staff writer at Live Science. He covers physics and astronomy, among other topics like tech and climate change. He graduated from University College London with a degree in particle physics before training as a journalist. When he's not writing, Ben enjoys reading literature, playing the guitar and embarrassing himself with chess.
Most Popular
By Harry Baker
By Ben Turner
By Jamie Carter