Largest objects ever get cooled down to their ‘quantum limit’
Physicists are bringing large objects closer than ever to the quantum realm
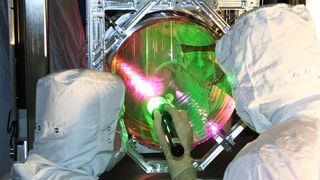
Physicists working at the world’s largest gravitational wave observatory have cooled their apparatus to near absolute zero in a bid to explore the so-called "quantum limit," or the point at which the rules that govern subatomic particles break down.
The purpose of this, according to the researchers, is not to study gravitational waves, but to understand why larger objects don't follow the rules of quantum mechanics, which some physicists believe may be because of the disruptive effects of gravity -- which may cause objects to decohere at large scales, so that they no longer quantum rules, but macroscopic ones instead.
In the new study, researchers at the Laser Interferometer Gravitational-Wave Observatory (LIGO) cooled four of the experiment’s mirrors, each weighing roughly 90 pounds (40 kilograms), from room temperature down to 77 nanokelvin (minus 459.6699998614 degrees Fahrenheit), reducing the vibrations of their atoms to just above the lowest level permitted by the rules of quantum mechanics.
Related: To hunt gravitational waves, scientists had to create the quietest spot on Earth
This achievement is an enormous leap in the size of an object that can be cooled to this extent. So far, the biggest thing to have been completely cooled to its lowest possible energy level — or ground state — is a tiny 150-nanometer (6x10 ^ minus 6 inches) wide glass bead weighing just a few fractions of a gram.
"Nobody has ever observed how gravity acts on massive quantum states," project director Vivishek Sudhir, assistant professor of mechanical engineering at MIT, said in a statement. "We’ve demonstrated how to prepare kilogram-scale objects in quantum states. This finally opens the door to an experimental study of how gravity might affect large quantum objects, something hitherto only dreamed of."
Physics is still unable to describe how gravity works at subatomic scales, leaving physicists scratching their heads when it comes to understanding the singularities that lie in the centers of black holes, or why gravity is so much weaker in strength than all of the other fundamental forces of nature (electromagnetism, weak force and strong force). Gravity is instead only described by our best theory of very large objects, Einstein's general theory of relativity. But since this theory breaks down at small scales, it leaves scientists with a fractured picture of how the universe works.
To observe the miniscule effects of gravity on a large-scale object, all possible outside noise — which is anything that can interfere with the signal they want to find, here it’s the random jostling of molecules — must be removed from the system, and that means making it incredibly cold. The temperature of an object and the amount it vibrates are one and the same thing. So cooling anything to absolute zero means removing all of the quantum-scale packets of vibrations, called phonons, that move through it.
To remove these vibrations, the LIGO team shone extremely precise lasers on the mirrors to measure the mirrors’ vibrations before using an electromagnetic field to apply a force opposing the mirrors’ motion, slowing them down and extinguishing the majority of tiny vibrations across them. In this way, they were able to reduce the average number of phonons at any given time in the system from 10 trillion to 10.8.
Now that they’ve removed most of the vibrations from their four-mirror system, the physicists want to study the mirrors’ quantum states to see how large-scale objects go about losing their quantum properties, a process called decoherence.
This isn’t the first experiment to have probed quantum effects in the macroscopic world. In May 2021, a team was able to observe quantum entanglement in a pair of near macro-scale drums, about 10 micrometers long, Live Science previously reported. In March 2021, Live Science also reported on the tiniest measurement of a gravitational force ever made, another attempt to delve deeper into gravity’s behavior on quantum scales.
The researchers published their findings June 18 in the journal Science.
Originally published on Live Science.
Live Science newsletter
Stay up to date on the latest science news by signing up for our Essentials newsletter.
Ben Turner is a U.K. based staff writer at Live Science. He covers physics and astronomy, among other topics like tech and climate change. He graduated from University College London with a degree in particle physics before training as a journalist. When he's not writing, Ben enjoys reading literature, playing the guitar and embarrassing himself with chess.
Most Popular
By Harry Baker
By Ben Turner
By Jamie Carter