Does reality exist when we're not looking?
In quantum physics, particles exist in many states at once until you measure them. Can reality really work that way?
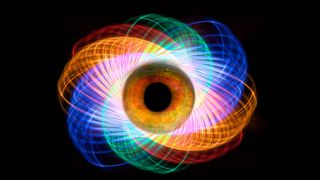
The standard interpretation of quantum mechanics places a lot of emphasis on the act of measurement. Before measurement, quantum systems exist in many states at once. After measurement, the system "collapses" into a specific value, so it's natural to ask what's really going on when measurements don't take place. There isn't a clear answer, and different ideas can go in some really wild directions.
One of the first lessons that physicists learned when they started examining subatomic systems in the early 20th century was that we do not live in a deterministic universe. In other words, we cannot precisely predict the outcome of every experiment.
For example, if you shoot a beam of electrons through a magnetic field, half of the electrons will curve in one direction while the other half will curve in the opposite direction. While we can build mathematical descriptions of where the electrons go as a group, we cannot say which direction each electron will take until we actually perform the experiment.
In quantum mechanics, this is known as superposition. For any experiment that can result in many random outcomes, before we make a measurement, the system is said to be in a superposition of all possible states simultaneously. When we make a measurement, the system "collapses" into a single state that we observe.
The tools of quantum mechanics are there to make some sense out of this chaos. Instead of giving precise predictions for how a system will evolve, quantum mechanics tells us how superposition (which represents all the various outcomes) will evolve. When we make a measurement, quantum mechanics tells us the probabilities of getting one outcome over another.
And that's it. Standard quantum mechanics is silent as to how this superposition actually works and how measurement does the job of collapsing the superposition into a single result.
Schrödinger's cat
If we take this line of thinking to its logical conclusion, then measurement is the most important act in the universe. It transforms fuzzy probabilities into concrete results and changes an exotic quantum system into verifiable results that we can interpret with our senses.
But what does that mean for quantum systems when we're not measuring them? What does the universe really look like? Does everything exist but we are simply unaware of it, or does it not really have a defined state until measurement takes place?
Ironically, Erwin Schrödinger, one of the founders of quantum theory (it's his equation that tells us how the superposition will evolve in time), railed against this line of thinking. He developed his famous cat-in-a-box thought experiment, now known as Schrödinger's cat, to show how ridiculous quantum mechanics was.
Here's a highly simplified version. Put a (live) cat in a box. Also put in the box some sort of radioactive element that is tied to the release of a poisonous gas. It doesn't matter how you do it; the point is to introduce some ingredient of quantum uncertainty into the situation. If you wait awhile, you won't know for sure if the element has decayed, so you won't know if the poison has been released and thus if the cat is alive or dead.
In a strict reading of quantum mechanics, the cat is neither alive nor dead at this stage; it exists in a quantum superposition of both alive and dead. Only when we open the box will we know for sure, and it's also the act of opening the box that allows that superposition to collapse and the cat to (suddenly) exist in one state or the other.
Schrödinger used this argument to express his astonishment that this could be a coherent theory of the universe. Are we really to believe that until we open the box that the cat doesn't really "exist" — at least in the normal sense that things are always definitely alive or dead, not both at the same time? To Schrödinger, this was too far, and he quit working on quantum mechanics shortly thereafter.
Decoherence
One response to this bizarre state of affairs is to point out that the macroscopic world does not obey quantum mechanics. After all, quantum theory was developed to explain the subatomic world. Before we had experiments that revealed how atoms worked, we had no need for superposition, probabilities, measurement or anything else quantum-related. We just had normal physics.
So it doesn't make sense to apply quantum rules where they don't belong. Niels Bohr, another founder of quantum mechanics, proposed the idea of 'decoherence" to explain why subatomic systems obey quantum mechanics but macroscopic systems do not.
In this view, what we understand as quantum mechanics is true and complete for subatomic systems. In other words, things like superposition really do happen for tiny particles. But something like a cat in a box is most definitely not a subatomic system; the cat is made of trillions of individual particles, all constantly wiggling, colliding and jostling.
Every time two of those particles bump into each other and interact, we can use quantum mechanics to understand what goes on. But once a thousand, or a billion, or trillions upon trillions of particles enter the mix, quantum mechanics loses its meaning — or "decoheres" — and regular macroscopic physics takes its place.
In this view, a single electron — but not a cat — in a box can exist in an exotic superposition.
However, this story does have limitations. Most important, we have no known mechanism for translating quantum mechanics into macroscopic physics, and we can't point to a specific scale or situation where the switch takes place. So, even though it sounds good on paper, this model of decoherence doesn't have a lot of firm backing.
So does reality exist when we're not looking? The ultimate answer is that it appears to be a matter of interpretation.
Live Science newsletter
Stay up to date on the latest science news by signing up for our Essentials newsletter.
Paul M. Sutter is a research professor in astrophysics at SUNY Stony Brook University and the Flatiron Institute in New York City. He regularly appears on TV and podcasts, including "Ask a Spaceman." He is the author of two books, "Your Place in the Universe" and "How to Die in Space," and is a regular contributor to Space.com, Live Science, and more. Paul received his PhD in Physics from the University of Illinois at Urbana-Champaign in 2011, and spent three years at the Paris Institute of Astrophysics, followed by a research fellowship in Trieste, Italy.
Most Popular
By Harry Baker
By Ben Turner
By Jamie Carter