Thousands of California worms wriggle into super blobs
Blackworm blobs don't need a leader to get to where they're going.
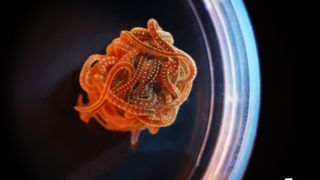
It wriggles. It squirms. It moves like some sort of multi-tentacled horror from the black lagoon. It's … a blob of blackworms. And just in time for Halloween!
California blackworms (Lumbriculus variegatus) are a species of unassuming aquatic worm that typically grow no more than about 1.5 inches (4 centimeters) long. But when threatened by environmental stressors — such as drought — these worms braid themselves together into masses to preserve moisture and protect one another. That's creepy enough, but these masses can also move in a form of what researchers call "emergent locomotion." No one's in charge, but the worm blobs can still steer themselves to more comfortable environments simply by dint of each worm's interactions with their nearest neighbors.
Now, researchers have figured out that the worms pull this off with a carefully calibrated mix of wiggliness and clinginess.
"We found there is a very fine balance required," said Chantal Nguyen, a postdoctoral researcher at the BioFrontiers Institute of the University of Colorado Boulder.
The results could be used to develop soft, swarm-like robotics with many small, simple parts that work together.
Related: Bizarre 'worm tornado' in New Jersey has scientists baffled
Blob of worms
Lots of different kinds of worms cluster together for safety when their environment becomes hostile (take a peek at any composting blog for more on earthworm "balling"). But few are documented to move as one when they're in these clusters. California blackworms can mass together by the thousands, though, and the resulting blobs seem to have a mind of their own, according to research published in February in the journal Proceedings of the National Academy of Sciences. That research found that blobs of blackworms essentially act like a non-Newtonian fluid, or a fluid that changes thickness depending on the amount of stress it's under. (The classic kitchen concoction of this fluid involves a mixture of cornstarch and water, which feels solid if you squeeze it suddenly and liquid if you run a finger through it slowly.) In other words, a whole bunch of worms clinging tightly to each other act a bit like a solid, but if they loosen up a bit, they're kind of like a liquid.
Nguyen joined the Georgia Tech researchers Yasemin Ozkan-Aydin and M. Saad Bhamla, who led that study, in order to model the movement of these worm blobs.
"It looks really cool to see just this giant blob of these worms kind of sliding around," Nguyen said. She had been working on modeling collective systems, so the opportunity to apply that work to the worms seemed intriguing.
First, the research team conducted experiments on individual worms to see how they moved in different water temperatures. This was to gather real-world worm movement data into their eventual computer model. In water of 86 degrees Fahrenheit (30 degrees C) or lower, the worms were prone to explore. They typically set out in a straight line until they hit the wall of the dish they were in, and then nosed around the edge. Above 86 F, the worms coiled up and moved about very little. Temperatures of over 93.2 F (34 C) proved dangerous — and eventually fatal — to the living worms.
Next, the researchers studied how real worm blobs reacted in different temperatures. At low temperatures of 50 F (10 C) and lower, the worms clung to each other in a tight mass. At 77 F (25 C), they relaxed a bit into a loose mass, but stayed together. At high temperatures near the edge of survivability, they quickly disentangled into individual coils.
Model worms
The researchers then used these behaviors to create a computer model of worms that could bend, self-propel and interact with one another. The model was in two-dimensions, not three, so it wasn't precisely representative of blackworm blobs, Nguyen said; — in deep enough water, the blobs can be spherical. But the researchers were able to find that a mixture of self-propelled wriggling and wormy clinginess was able to reproduce the kind of motion seen in real worm blobs. The researchers created a temperature gradient in their virtual worm world so that one side of the model worm-blob enclosure was cooler than the other. They first simulated a single worm and found that the automatic movements of the worm in different temperatures led to the worm "finding" the cool side: In cooler waters, the worms straightened out and crawled forward, creating a sort of feedback loop such that the cooler the enclosure was, the more the worm could direct its movement straight ahead.
The researchers then simulated a worm blob. They found that the blobs, too, tended to move to cooler waters. But to do so, they had to wiggle just enough to move without breaking apart their congregation.
"Only for a very fine balance between the active forces and the attraction between worms were we able to see the worm blob move as a collective from the hot to the cold," Nguyen said.
The next step is to make the model 3D, Nguyen said, and then to start developing robots based on the worms' weird movements. The robotics field has a lot of interest in swarm robots, which are simple individual robots that interact with one another to complete more complex tasks than they could do on their own. There is also a lot of interest in soft robots inspired by nature. Thanks to their pliability and flexibility, soft robotics are promising technology for biomedicine, Nguyen said. The worm blobs combine both swarm robotics and soft robotics, she said.
"A lot of current swarm robotic systems consist of rigid elements," she said, "and thus soft swarm robotics is very much an open field of research."
The findings appeared Sept. 30 in the journal Frontiers in Physics.
Originally published on Live Science.
Editor's note: This article was updated to state that some of the researchers are from Georgia Tech, not the University of Georgia as had been previously stated.
Live Science newsletter
Stay up to date on the latest science news by signing up for our Essentials newsletter.
Stephanie Pappas is a contributing writer for Live Science, covering topics ranging from geoscience to archaeology to the human brain and behavior. She was previously a senior writer for Live Science but is now a freelancer based in Denver, Colorado, and regularly contributes to Scientific American and The Monitor, the monthly magazine of the American Psychological Association. Stephanie received a bachelor's degree in psychology from the University of South Carolina and a graduate certificate in science communication from the University of California, Santa Cruz.
Most Popular
By Harry Baker
By Ben Turner
By Jamie Carter